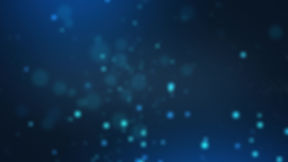
Effects of Hormones on Midshipman Acoustic Communication
Seasonal Variation in Circulating Hormone Levels
The reproductive behavior discussed in Life History is mediated by changes in circulating steroid hormones such as testosterone, 11-ketotestosterone (11-KT), and estrogen. These shifts occur at certain periods in the midshipman fish’s life cycle, which is broken down into four different categories. The non-reproductive period from December to February is characterized by low GSI and their presence in the deep-water benthos (Sisneros et al., 2004b). From March to April, the midshipman fish migrate to the shallow depths offshore and gonadal recrudescence occurs, which comprises the pre-nesting period (Sisneros et al., 2004b). During the nesting period in June and July, fish are found in nests with maturing offspring (Sisneros et al., 2004b). Finally, in September during the post-nesting phase, they return to deeper depths with regressed gonads (Sisneros et al., 2004b).
During the pre-nesting period, females experience a single peak in both testosterone and estrogen, which activates the egg maturation process (Sisneros et al., 2004b). At all other periods of time, testosterone and estrogen are low (Sisneros et al., 2004b). Type I males, however, experience a peak in 11-KT that begins in the pre-nesting period and continues into the early nesting period while the male is calling (Sisneros et al., 2004b). When the male switches over to parental care, 11-KT drops to basal levels (Knapp et al., 1999; Sisneros et al., 2004b).
It is hypothesized that these shifts in hormone are potentially induced by changes in light cycle. A potential mechanism for this was discovered when gonadotropin-releasing hormone mRNA was found in neurons of the ventrolateral thalamic nucleus (Foran et al., 1997). This nucleus is modulated by retinal input and projects back to the retina, which could modulate the visual system in response to season changes in environmental light conditions (Foran et al., 1997).
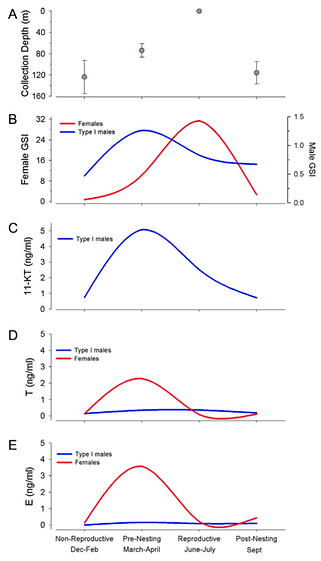
Plainfin midshipman were collected from Monterey Bay and Tomales Bay, CA and their plasma steroid concentrations were measured. (A) Collection depth and (B) gonadosomatic index (GSI) were taken as well. Specifically, (C) 11-keto-testosterone (11-KT), (D) testosterone (T), (E) estrodiol (E) were sampled through out the season. Modified from Sisneros (2004b).
Hormone Influence on the Vocal System and Behavior
Results from field studies have provided evidence for the vocal motor pathway’s dependence on steroid hormones. Non-calling Gulf toadfish, which are closely related to plainfin midshipman, begin to call and have elevated 11-KT when placed into a population of calling Gulf toadfish (Remage-Healey & Bass, 2005). In addition, if 11-KT is administered via food, toadfish call quantity increases within 10 to 20 minutes (Remage-Healey & Bass, 2006). These findings led to studies in the lab to understand the molecular processes by which hormones can influence calling behavior.
At the sonic muscle level, an increase in circulating steroid has obvious effects on muscle mass. With the increase in circulating androgen levels there is also an increase in sonic muscle mass (Sisneros et al. 2009)

Type I midshipman male with his developing offspring. Photo by Margaret Marchaterre.

Power spectrum of a typical type I male hum. The fundamental frequency of this hum is at 102 Hz. The next few harmonics have a similar or larger amplitude compared to the fundamental suggesting that they potentially carry valuable information for the female.
To search for the mechanism of these effects, Genova and team (2013) compared expression of androgen receptors in non-humming and humming type I males. Non-humming males exhibited higher ARβ mRNA in their sonic muscle while humming males had higher ARα mRNA in their sonic muscle (Genova et al., 2013). This indicates that the expression of ARβ plays a preparatory role while ARα plays a supportive role in the vocal muscle physiology during advertisement calling (Genova et al., 2013).
Moving to the circuitry, it was hypothesized that the priming of the vocal motor system is an interaction between androgen receptors and aromatase. Testosterone was found to upregulate aromatase mRNA in the vocal motor nucleus where ARβs are located (Bass & Forlano, 2008, Forlano et al., 2010). On a broader scale, a pilot study showed that implanting testosterone into juvenile male midshipman increased the vocal motor nucleus size and increased excitability of the vocal motor system overall (Bass & Forlano, 2008). The effects of 11-KT were also examined and found to rapidly potentiate call duration in type I males (Remage-Healey & Bass, 2006).
Time of Day Influence on Vocal Motor Circuitry
Another key factor that influences type I male calling is time of day. As stated previously, midshipman fish typically call during the night. Unfortunately, most experiments to investigate the vocal motor system were being conducted in the day. Feng and Bass (2014) found that the neural excitability of the vocal motor system increased in the dark. Furthermore, putting the type I males in five days of constant darkness drastically increased the vocal excitability when compared to males in five days of constant light (Rubow & Bass, 2009).
Since time of day seemed to affect the activity of the circuitry, it was hypothesized that melatonin was influencing the circuit in some way. Feng and Bass (2014) found that they could reverse the suppression of vocal excitability produced by five days of light by administering melatonin. Furthermore, they found that injecting a melatonin antagonist could reverse the excitability produced by housing a male in five days of darkness (Feng & Bass, 2014).

Summary of results supporting the role of melatonin in the nocturnal rise in vocal excitability among type I males in reproductive state. Vocal excitability was measured in terms of the minimum current of midbrain electrical stimuli needed to evoke a fictive call. Males received an implant of either 2-iodomelatonin (2-IMel), melatonin analogue or sesame oil (implant control, CON). Some males were kept 5 days in the dark (5DD) and others in the light (5LL). Adopted from Feng and Bass (2014).
Steroid-dependent Plasticity of Auditory Sensitivity
All these results supported the hypothesis that the excitability of the vocal motor system during the reproductive season in type I males is mediated partly by melatonin (Forlano et al., 2015b).
Since the perception of hums is vital for females to mate, it was hypothesized that their hearing must be tuned to the fundamental frequency of the hum. While exploring this hypothesis, Sisneros and Bass (2003) found that there was a difference between the tuning curves of females in and out of the breeding season. Reproductive female saccular afferents exhibited higher phase locking accuracy at 180 to 400 Hz, which are dominant frequencies in the male hum, than non-reproductive female saccular afferents (Sisneros & Bass, 2003). Subsequent studies have found that males undergo a similar change (Rohmann & Bass, 2011). Coffin and team (2012) found that this shift in hearing sensitivity occurred at the same time as an increase in hair cell density in the saccule. To further understand what was causing the increase in hair cell density, they also found that it correlated with a decrease in hair cell death and increase in the number of small, potentially immature hair cell bundles (Coffin et al., 2012). This combined with expression of steroid receptors in the saccule point to steroid hormones acting as a hair cell protectant.

In order to solidify the hypothesis that this change was mediated by steroid hormone shifts, Sisneros (2004a) placed testosterone and estrogen implants into non-reproductive females that had their ovaries removed. When these females were tested, they displayed a tuning curve similar to that of reproductive females with an increase in sensitivity to the dominant frequencies in a hum especially at higher frequencies (Sisneros et al., 2004a). It is hypothesized that this shift is critical to localizing males, because lower frequencies in the midshipman call are attenuated by the shallow rocky substrate that they nest in (Sisneros et al., 2004a).


Iso-intensity curves based on vector strength (VS) of synchronization. VS values shown for each frequency tested in terms of the median. Adopted from Sisneros & Bass (2003).
Combined plot of the phase-locking precision of saccular afferents in vector strength of synchronization (VS) and the power (amplitude) spectrum of a hum. There is an overlap between the testosterone-treated (triangles) and 17β-estradiol-treated (squares) nonreproductive females and wild-type reproductive females. Untreated wild-type nonreproductive females are shown as circles. Adapted from Sisneros et al. (2004a).
BK Channel Influence on the Observed Seasonal Shift in Hearing Sensitivity

Schematic summarizing proposed role of slo1 and BK abundance in seasonal auditory plasticity. Increased expression leads to robust encoding of higher frequencies in upper harmonics of advertisement calls. Adapted from Fettiplace and Fuchs (1999).
A molecular mechanism that explains this shift in hearing is increased expression of BK channels. These are large potassium current channel that are activated by changes in membrane potential or high calcium levels (Rohmann et al., 2013). In mammalian models, BK channels are known to increase the inherent firing frequency of the hair cell therefore allowing for better phase locking at higher frequencies (Rohmann et al., 2013). In addition, the expression of the slo1 gene, which forms BK channels is known to be regulated by reproductive state (Rohmann et al., 2013). Rohmann and team (2013), using absolute quantitative real-time PCR, found that both slo1 transcripts are upregulated in the saccule of reproductive females compared to non-reproductive females. To guarantee that the change in BK channels was responsible for the seasonal shift, they applied BK channel antagonists. Both the general potassium channel blocker, tetraethylammonium chloride, and the BK channel specific antagonist, iberiotoxin, shifted thresholds of reproductive females to the range of non-reproductive females (Rohmann et al., 2013). Beyond general shifts in hearing sensitivity, it was also found that individual differences in hearing sensitivity could be explained by differences in slo1 expression (Rohmann et al., 2013).
Dopaminergic Modulation of Auditory Sensitivity via Efferents from the Diencephalon
Another potential mechanism for this seasonal plasticity is via dopaminergic innervation of the peripheral auditory system. Forlano and team (2014) found via tract-tracing combined with immunohistochemistry that dopaminergic terminals originating from the periventricular posterior tuberculum innervate the saccule. These dopaminergic neurons also appear to project to numerous auditory nuclei including the octavolateralis efferent nucleus, hindbrain octaval nuclei, and auditory thalamus (Forlano et al, 2014; Perelmuter and Forlano, 2017). The discovery of robust aromatase mRNA and protein, ERα and ARβ

A
B
C
(A) Tyrosine hydroxylase (TH)-ir cells labelled green in the periventricular posterior tuberculum (TPp) just medial to the medial forebrain bundle and lateral and dorsal to the paraventricular organ. Cells labelled with DAPI in blue. (B) Three neurobiotin labeled-neurons after a saccular backfill. (C) Overlay of images in A and B. Adapted from Forlano et al. (2014).
mRNA, and ERβ2 protein in the periventricular posterior tuberculum make it a prime target for steroid action (Forlano et al., 2001; 2005; 2010). In addition, during the reproductive summer, the density and size of these dopaminergic terminals in the saccule significantly decreases (Forlano et al., 2015a). If these fibers are inhibitory in nature, the release of inhibition results in increased hearing sensitivity.
Modulation of Social and Reproductive Behaviors
Increased transduction of the hum at the level of the periphery is not enough to drive reproductive behavior. In order for reproduction to occur, a female must be able to distinguish the male hum from other noises and also be motivated to swim toward the hum. While not much is known about the higher order processing that occurs to allow for the midshipman to differentiate noises, one study sought to shed light on the matter. Looking at cFos as a proxy for brain activation, Mohr and team (2018) found that there was higher cFos activation at the levels of the diencephalic forebrain (central posterior nucleus in the auditory thalamus and anterior tuberal nucleus of the hypothalamus) during exposure to a conspecific hum compared to exposure to heterospecific calls or ambient noise. However, there was no difference between cFos activation in each exposure group when looking at the hindbrain (descending octaval nucleus and secondary octaval nucleus). This points towards circuitry at the level of the diencephalon being critical in identifying conspecific hums.
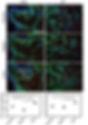
cFos-ir (red) activation is shown in these representative photomicrographs of the forebrain central posterior nucleus of the thalamus (CP) and the anterior tuberal nucleus of the ventral hypothalamus (AT). Neurons are identified by the presence of both the neuron specific anti-Hu (green) and the nuclear specific counterstain DAPI (blue). Fish were assigned one of three experimental exposure conditions: ambient noise (control), white seabass calls (hetero) or conspecific advertisement calls (hum). Graphical data are presented at the average number of cFos-ir neurons per section for each of the experimental conditions. *p<0.05, ***p<0.001, scale bar = 100μm.
Finally, motivation plays a crucial role in reproductive behavior. Forlano and team (2017) sought to explore the circuitry activated in females motivated to locate a simulated male advertisement call. This circuitry likely includes the social decision making network comprised of the mesolimbic reward system and social behavior network (O’Connell and Hofmann, 2011, 2012) , which included hormone sensitive

Representative photomicrographs of the periventricular posterior tuberculum (TPp) for both low and high response time. DAPI (blue) identify all neurons. cFos-ir (red, arrowheads) stained cells signify activation. Tyrosine hydroxylase (TH) cells labelled green show production of dopamine. More activation can be observed in the high response time animal. Adopted from Forlano et al. (2017).
nuclei in the basal forebrain and midbrain (Goodson, 2005). By using phonotaxis as an indication of motivation to reproduce, Forlano and team (2017) found that with increased time on a speaker producing the fundamental frequency of a hum there was a positive correlation with activation in the ventromedial and ventrolateral thalamic nucleus, periventricular posterior tuberculum, preoptic nuclei, and dorsomedial telencephalon. Both the ventromedial and ventrolateral thalamic nucleus and periventricular posterior tuberculum are dopaminergic and innervate auditory nuclei throughout the brain (Forlano et
al., 2017). The preoptic nucleus is a major endocrine and sensory motor integration center that controls reproduction and homeostatic behavior (Butler & Hodos, 2005). The fact that it receives input from the ascending auditory system make it a likely driver for phonotaxis (Bass et al., 2000). Even more proof of this is that in anurans lesioning of the preoptic area results in the inability to phonotax (Walkowiak et al., 1999). Finally, the dorsomedial telencephalon represents the highest level of auditory processing in fish (McCormick 2011) and projects to social behavior network nuclei such as ventral tuberal and anterior hypothalamus (O’Connell & Hoffmann, 2011; 2012). Activation of these nuclei during phonotaxis behavior indicate their role in motivating females to reproduce.
References
Bass, A. H., & Forlano, P. M. (2008). Neuroendocrine mechanisms of alternative reproductive tactics: the chemical language of reproductive and social plasticity. Alternative Reproductive Tactics. An Integrative Approach, 116-120.
Butler, A. B., & Hodos, W. (2005). Comparative vertebrate neuroanatomy: evolution and adaptation. John Wiley & Sons.
Coffin, A. B., Mohr, R. A., & Sisneros, J. A. (2012). Saccular-specific hair cell addition correlates with reproductive state-dependent changes in the auditory saccular sensitivity of a vocal fish. Journal of Neuroscience, 32(4), 1366-1376.
Feng, N. Y., & Bass, A. H. (2014). Melatonin action in a midbrain vocal-acoustic network. Journal of Experimental Biology, 217(7), 1046-1057.
Forlano, P. M., Deitcher, D. L., & Bass, A. H. (2005). Distribution of estrogen receptor alpha mRNA in the brain and inner ear of a vocal fish with comparisons to sites of aromatase expression. Journal of Comparative Neurology, 483(1), 91-113.
Forlano, P. M., Deitcher, D. L., Myers, D. A., & Bass, A. H. (2001). Anatomical distribution and cellular basis for high levels of aromatase activity in the brain of teleost fish: aromatase enzyme and mRNA expression identify glia as source. Journal of Neuroscience, 21(22), 8943-8955.
Forlano, P. M., Kim, S. D., Krzyminska, Z. M., & Sisneros, J. A. (2014). Catecholaminergic connectivity to the inner ear, central auditory, and vocal motor circuitry in the plainfin midshipman fish Porichthys notatus. Journal of Comparative Neurology, 522(13), 2887-2927.
Forlano, P. M., Licorish, R. R., Ghahramani, Z. N., Timothy, M., Ferrari, M., Palmer, W. C., & Sisneros, J. A. (2017). Attention and motivated response to simulated male advertisement call activates forebrain dopaminergic and social decision-making network nuclei in female midshipman fish. Integrative and comparative biology, 57(4), 820-834.
Forlano, P. M., Marchaterre, M., Deitcher, D. L., & Bass, A. H. (2010). Distribution of androgen receptor mRNA expression in vocal, auditory, and neuroendocrine circuits in a teleost fish. Journal of Comparative Neurology, 518(4), 493-512.
Forlano, P. M., Sisneros, J. A., Rohmann, K. N., & Bass, A. H. (2015b). Neuroendocrine control of seasonal plasticity in the auditory and vocal systems of fish. Frontiers in neuroendocrinology, 37, 129-145.
Genova, R. M., Marchaterre, M. A., Knapp, R., Fergus, D., & Bass, A. H. (2012). Glucocorticoid and androgen signaling pathways diverge between advertisement calling and non-calling fish. Hormones and behavior, 62(4), 426-432.
Goodson JL (2005). The vertebrate social behavior network: evolutionary themes and variations. Hormones and behavior, 48, 11–22.
McCormick, C. A. (2011). HEARING AND LATERAL LINE| Auditory/Lateral Line CNS: Anatomy.
Mohr, R. A., Chang, Y., Bhandiwad, A. A., Forlano, P. M., & Sisneros, J. A. (2018). Brain Activation Patterns in Response to Conspecific and Heterospecific Social Acoustic Signals in Female Plainfin Midshipman Fish, Porichthys notatus. Brain, behavior and evolution, 91(1), 31-44.
O'Connell, L. A., & Hofmann, H. A. (2011). The vertebrate mesolimbic reward system and social behavior network: a comparative synthesis. Journal of Comparative Neurology, 519(18), 3599-3639.
O’Connell, L. A., & Hofmann, H. A. (2012). Evolution of a vertebrate social decision-making network. Science, 336(6085), 1154-1157.
Remage-Healey, L., & Bass, A. H. (2005). Rapid elevations in both steroid hormones and vocal signaling during playback challenge: a field experiment in Gulf toadfish. Hormones and Behavior, 47(3), 297-305.
Remage-Healey, L., & Bass, A. H. (2006). From social behavior to neural circuitry: steroid hormones rapidly modulate advertisement calling via a vocal pattern generator. Hormones and Behavior, 50(3), 432-441.
Rohmann, K. N., & Bass, A. H. (2011). Seasonal plasticity of auditory hair cell frequency sensitivity correlates with plasma steroid levels in vocal fish. Journal of Experimental Biology, 214(11), 1931-1942.
Rohmann, K. N., Fergus, D. J., & Bass, A. H. (2013). Plasticity in ion channel expression underlies variation in hearing during reproductive cycles. Current Biology, 23(8), 678-683.
Rubow, T. K., & Bass, A. H. (2009). Reproductive and diurnal rhythms regulate vocal motor plasticity in a teleost fish. Journal of Experimental Biology, 212(20), 3252-3262.
Sisneros, J. A., Alderks, P. W., Leon, K., & Sniffen, B. (2009). Morphometric changes associated with the reproductive cycle and behaviour of the intertidal‐nesting, male plainfin midshipman Porichthys notatus. Journal of fish biology, 74(1), 18- 36.
Sisneros, J. A., & Bass, A. H. (2003). Seasonal plasticity of peripheral auditory frequency sensitivity. Journal of Neuroscience, 23(3), 1049-1058.
Sisneros, J. A., Forlano, P. M., Deitcher, D. L., & Bass, A. H. (2004a). Steroid-dependent auditory plasticity leads to adaptive coupling of sender and receiver. Science, 305(5682), 404-407.
Sisneros, J. A., Forlano, P. M., Knapp, R., & Bass, A. H. (2004b). Seasonal variation of steroid hormone levels in an intertidal-nesting fish, the vocal plainfin midshipman. General and comparative endocrinology, 136(1), 101-116.
Walkowiak, W., Berlinger, M., Schul, J., & Gerhardt, H. C. (1999). Significance of forebrain structures in acoustically guided behavior in anurans. European journal of morphology, 37(0), 177-181.